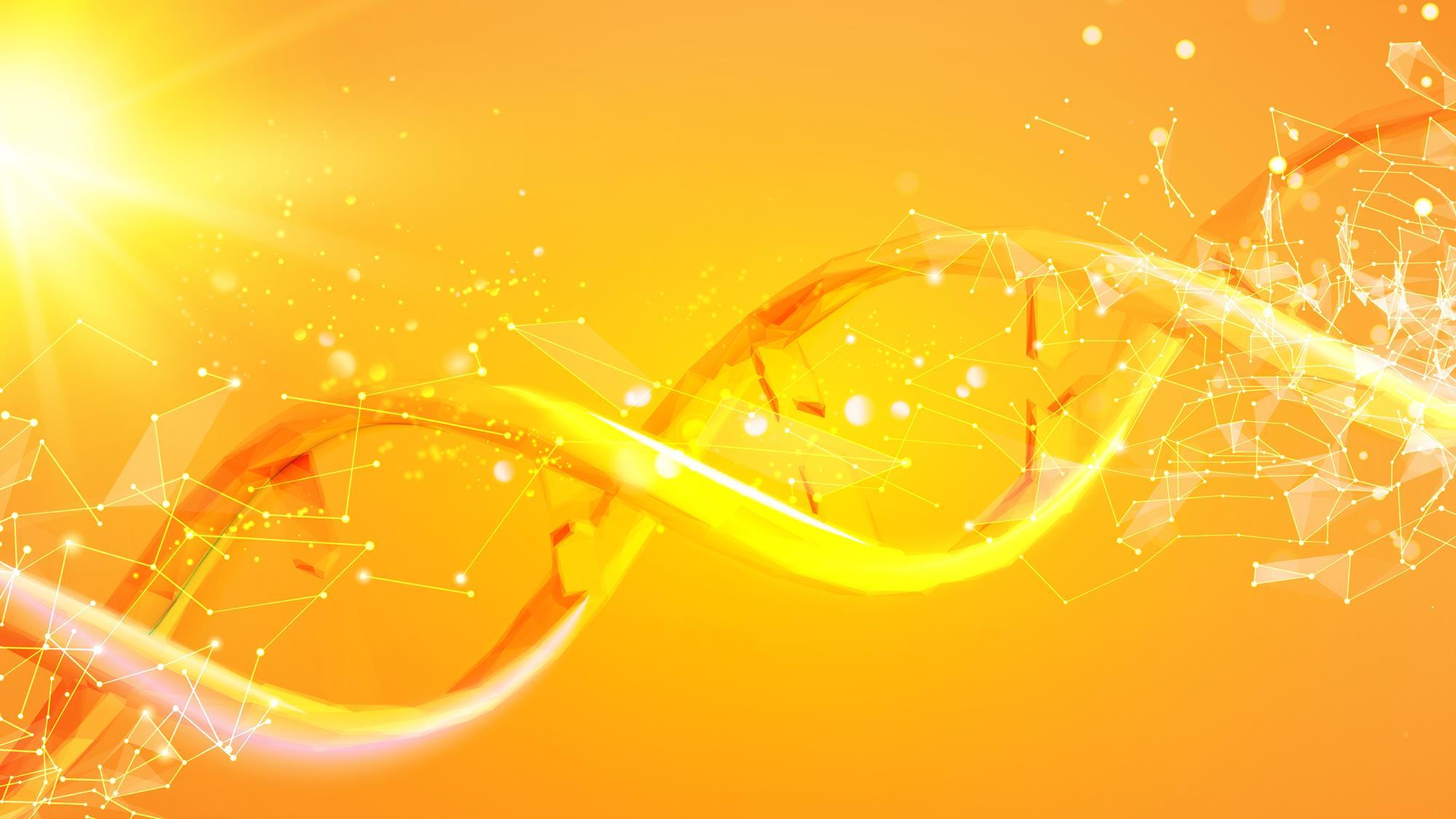
15 Aug HOW DOES UV LIGHT AFFECT OUR DNA?
What is UV Light?
Ultraviolet (UV) light is a type of electromagnetic radiation invisible to most humans and emitted mainly by the sun. However, UV can be released by artificial sources too such as electric arcs and specialized lights (e.g. tanning lamps). The wavelengths of UV rays range between 200 and 400 nm, which are shorter than the wavelengths of violet light (hence the name ultra-violet. Violet is at the higher end of the visible spectrum with a wavelength of around 380–450 nm).
How can UV affect our DNA?
DNA is our genetic code, the precious molecule containing all the information necessary to make up a living organism and it is therefore well protected inside a special structure, the nucleus, located deep inside each cell (Figure 1). DNA is composed of two complementary strands that are wound into a double helix (Figure 1). The hereditary message is chemically coded and made up of the four bases, the four ‘building blocks’: adenine (A), thymine (T), guanine (G) and cytosine (C ) (Figure 1).
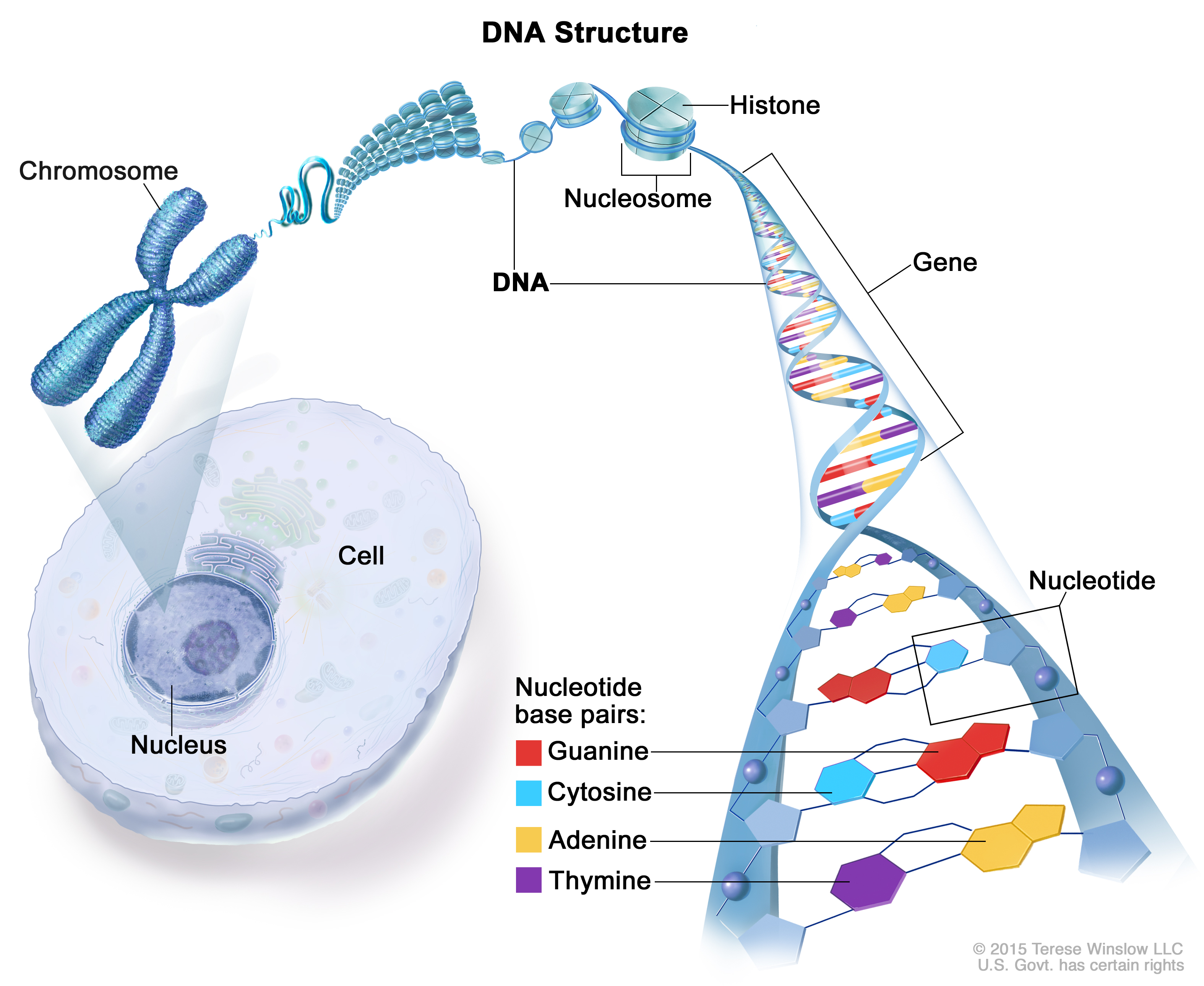
Unfortunately, UV can reach the nucleus and in particular DNA, causing many harmful effects. It all starts when a photon of UV light ‘hits’ DNA. DNA is a large molecule that absorbs the energy of the photon and then quickly releases that energy in the form of heat. After the DNA absorbs the energy and before it releases the heat, it is in a higher energy state and is therefore more reactive. The shorter the time that the DNA is in this ‘reactive state’ the less likely it is that it will undergo a harmful photo-reaction. It turns out that DNA is extremely effective at releasing the extra energy quickly, so it gets damaged less than 0.1% of the time it is being hit by UV light.
However, in the cases where damage does occur, how does it happen?
1. Direct damage: Fusion/ Dimerization of neighboring pyrimidine DNA bases (thymine or cytosine)
There are different ways UV-excited DNA can react, but the fusion or dimerization of two adjacent bases (the building blocks of the DNA) is the most common. If two pyrimidine bases (thymine or cytosine; two out of the four bases that make up the DNA backbone. The other two are adenine and guanine) are next to each other, their two rings can fuse together forming a covalent link (Figure 2). This type of reaction, called a pericyclic reaction, is possible because of how close their rings are and how their symmetries align (Figure 2). Such a chemical change to the DNA is not a mutation but is rather DNA damage or a DNA lesion. The DNA at a damaged site is no longer truly DNA as it has a different chemical structure to the natural form of DNA and it is a chemical intermediate. This formation of a four-carbon ring between the pyrimidines (see red square on Figure 2) creates a ‘notch’ on the DNA backbone causing problems for essential procedures happening along the DNA backbone.

For example, every time a new cell needs to be made, the DNA has to be replicated. This process happens when the double helix opens up to two single strands so that replication proteins can bind along the DNA backbone and start constructing a new strand according to the instructions they see on the original backbone. Every A base they come across ‘asks’ for a T base — as a pair — and vice versa, while every G they come across ‘screams’ for a C and vice versa. (This happens as A is chemically complementary to T, while G complements chemically C). Since the backbone is no longer ‘smooth’ due to the photoproduct of the pericyclic reaction, it is difficult for the replication proteins to determine what base pairs should be put across the fused, covalently-linked pyrimidines when the altered/ damaged DNA strand needs to be copied and a new DNA strand to be synthesized. This may result in a wrong base being put during the replication process (if the damage is not properly repaired on time). Exactly this base change resulting from the DNA lesion is called mutation.
A copying mistake like this can in turn change how the DNA encodes a protein, resulting in an abnormal protein. If the mutation occurs in an area which codes for DNA repair enzymes, tumor promoting and/ or tumor suppressing proteins, this mutation could even lead to cancer. For example, the UVB-induced lesions (UVB is the middle wave UV light, 280–315 nm) generate typical genetic mutations resulting from the incorrect change of cytosine to thymine base (C → T and CC → TT transitions), called the ‘UVB signature’ mutations. Such mutations are commonly found in the TP53 tumor suppressing gene in non-melanoma skin cancers (such as Basal Cell Carcinomas — BCCs).
2. Indirect damage: Oxidative Stress
UV exposure doesn’t always lead directly to mutations in the DNA. In fact, UVA (long wave UV light, 315–400 nm) radiation commonly causes the creation of a free radical that then interacts with and oxidizes DNA bases. A free radical is created when oxygen (normally found in the form of two bound atoms O2) in the body splits into single atoms with unpaired electrons. Electrons like to be in pairs, so these atoms, called free radicals, scavenge the body to seek out other electrons so they can become a pair.
UVA may hit a molecule (like an amino acid, the building block of proteins) which isn’t built to dissipate the energy acquired by the photon that hits it. When this happens, the excited molecule can excite an adjacent oxygen atom, turning the stable molecule into a reactive species. Oxygen is much less stable in its excited, higher energy state. This means it will react with any protein or lipid it collides within the cell in order to go back to its more stable, lower energy state. Although it can damage various molecules in the cell, the biggest damage occurs when it hits DNA. When an excited oxygen hits DNA, it can cause a G to T transversion. The oxidation of guanine, for example, prevents the hydrogen bonding required to base pair with cytosine. Instead, during replication, 8-oxoguanine (damaged guanine site by oxidation) can base pair with adenine via two hydrogen bonds. When the second strand is synthesized, the base position originally occupied by a guanine is then replaced with a thymine, leading to a G to T transversion.
As in the case of direct DNA damage, this mutation alters how the DNA is translated into a protein and can be potentially harmful. Part of what makes this type of DNA damage particularly dangerous is that it is caused by excited oxygen molecules, not the UV light itself. Excited oxygen has an unusually long lifespan for a reactive species, so the damage can occur in cells other than skin cells.
Is there any hope?
Our bodies do not simply ‘lie down and accept their fate’; there are numerous defense mechanisms to protect against and mitigate the damage. When direct DNA damage fuses two base pairs together, the DNA ends up with a bulge in its normal double helix shape. Several enzymes travel around the DNA guarding it and looking for such abnormalities. When they find such a bulge, an abnormal notch, they activate repair proteins that cut out the damaged part of the DNA and put in the correct base pairs. This whole process is called nucleotide excision repair. The effect of indirect DNA damage is harder to detect because transversion does not result in a distorted helix. The mechanism that repairs this kind of damage is called base excision repair. Enzymes called DNA glycosylases remove a base pair misplaced by transversion; other enzymes then open up the DNA’s backbone so that DNA building enzymes can come through and fill the gap with the correct base pair.
Last but not least, melanosomes and melanin play a major role too in our protection against UV damage. (Read more here)
Sorry, the comment form is closed at this time.